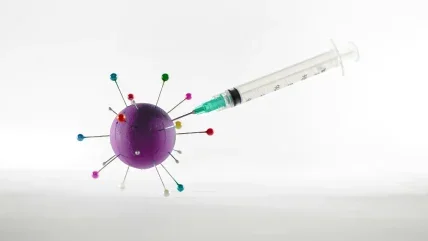
“If you didn’t have the nanoparticles,” says Robert Langer, MIT institute professor and Moderna co-founder, “you wouldn’t have the vaccines”. There’s no fastest development in history without the half-century saga of a family of engineered fats. Not a moment too soon, mRNA technology, lipid nanoparticles (LNPs) and bioinformatics have turned the pharmaceutical industry upside-down.
“It’s really amazing that the three technologies came in basically one timing,” says Dan Peer, director of the laboratory of precision nanomedicine at Tel Aviv University. “You bring together three different strategies into one product and, at the end, it could change the world.” Speaking from his office in Israel, where more than half of the population has been fully vaccinated – most with Pfizer-BioNTech’s Comirnaty – Peer means that in two senses. Already, mRNA vaccines are playing a vital part in tackling the pandemic, but in the longer term, the same technologies will enable drug development to take place over months rather than years and will result in far more targeted, far less toxic therapeutics with smaller manufacturing footprints.
“Almost everything is druggable at this point,” adds Peer, who, when he says, “we are actually living inside the revolution”, also means that there’s a revolution happening inside us. “Think of all those rare genetic diseases where you need to bring a protein: instead of bringing a new protein, now you can just generate it – it’s like there’s a factory inside your body.”
Pieter Cullis, head of the Nanomedicines Research Group at the University of British Columbia and co-founder of Acuitas, provider of LNPs for the Pfizer- BioNTech vaccine, expands on the same point. “Say you have cancer and you want to use an antibody that recognises the cancer, well, now, instead of building a big plant to make the antibody, you can just make the messenger RNA that codes for the antibody, put that into an LNP and have your liver make it,” he explains. “And in many ways, it’ll make a better version, because the ones that are made in these big plants are quite often a bit immunogenic as a result.”
This is what Cullis calls the “third generation” of pharmaceuticals. Thanks to LNPs, these “smart medicines” can both deliver small molecules more specifically to where they’re needed, and enable the therapeutic use of macromolecules like mRNA, siRNA and DNA, which would otherwise be destroyed by the body’s defence mechanisms.
“We desperately need to use larger RNA and DNA-based molecules, because then you can make use of your understanding of how cells actually work to silence, express or edit a gene.”
Pieter Cullis
“The reason we use small molecules so much is because they’re the only molecules that can get into cells to affect what’s going on inside,” he explains. “But we desperately need to use larger RNA and DNA-based molecules, because then you can make use of your understanding of how cells actually work to silence, express or edit a gene.” In the case of the mRNA Covid-19 vaccines, LNPs transfect synthetic RNA ‘instructions’ for producing the SARS-CoV-2 spike protein into cells, which respond by building the protein and displaying it on their surface. Other immune cells then recognise the foreign antigen and mount an adaptive immune response, preparing the body to fend off future infection. Outsourcing so much work to the recipient saves a huge amount of time: within months of the SARS-CoV-2 virus being sequenced, multiple mRNA vaccines were in the clinic.
“As soon as you know what protein it is that you want to express, or silence – or whatever, for that matter – then your medicine can effectively be ready in a couple of months,” continues Cullis. “All you have to do is synthesise the messenger RNA. The encapsulation procedure in these nanoparticles is very straightforward. And then you’ve got your potential very targeted therapeutic. It completely short-circuits your average small molecule development time, which is often 15 years and can cost a billion dollars.” Developing the right LNPs, however, has been an even longer and more arduous journey than that.
Walk through walls
Langer, a kind of nanotechnology genealogist on top of everything else, traces the LNP family tree back to Alec Bangham’s discovery and development of liposomes (originally ‘banghasomes’) for delivering small molecule drugs in the 1960s. A decade later, Langer himself took on the challenge of (and the derision for) using similarly tiny (polymer) particles to safely carry, and slowly release larger proteins and nucleic acids in order to test angiogenesis inhibitors for treating cancer. To many experts, what he was attempting was closer to magic than science. Getting a large molecule through a nanoparticle shell was like “walking through a brick wall”.
“I always tell the joke that I found 200 different ways to get it to not work, which is true,” he says. “And when I first did it, people were very sceptical. I got my first nine grants rejected and no chemical engineering department in the country would hire me.” Slowly, though, the world came to appreciate what Langer, incessantly tinkering with the tortuosity and porosity of his polymers, had made possible.
The next step on the similarly tortuous LNP pathway was to find a way to smuggle these large molecule delivery systems past the immune system they were designed to support, which Langer and others managed to do in the 1990s, by adding polyethylene glycol (PEG) to some of the lipids in nanoparticles. Pegylated lipids are one of the four ingredients in the LNPs used in today’s mRNA vaccines, keeping particles regular through the formulation process and preventing them from aggregating in storage, as well as disguising them from the immune system.
Cullis, who began encasing cancer drugs in liposomes in the 1980s and has often been dubbed the ‘godfather’ of LNPs, enters Langer’s tale in the following decade, when one of his companies, Inex Pharmaceuticals, made a breakthrough on the next ingredient. One of the reasons nucleic acids, which carry a negative electric charge, need to be incorporated into delivery vehicles is that they can’t cross our cells’ similarly negatively charged lipid membranes. You may have spotted a problem there. There are no negatively charged lipids in nature, so synthetic, positively charged ones needed to be engineered before LNPs could even pick up their payloads. This was first done in the 1980s, but these ‘cationic’ lipids didn’t so much cross cell membranes as destroy them. So, through the late 1990s and early 2000s, Inex and its sister company Protiva Therapeutics developed ‘ionisable’ lipids that were positively charged at a low pH, but were neutral in the blood.
Nothing comes easy on the nanoscale, so, of course, the first versions of these ‘ionisable cationic lipids’ were still toxic, but just as Langer had done in the 1970s, Inex and others strenuously tweaked, modified and optimised them, casting hundreds aside in the process. Their work eventually resulted in the LNP formulation used in Alnylam’s Onpattro, which became the first RNA drug to reach the market in 2018. The process Cullis and his colleagues developed for combining ionisable lipids with nucleic acids also laid the blueprints for today’s LNP manufacturing processes. In “cartoon form”, they found that rapidly mixing lipids in ethanol with nucleotides in water at roughly pH 4 causes the ingredients to bind as they fall out of the solution. “At the end of it, all that’s left are these little globules that are stabilised by the PEG lipids,” explains Cullis. “You just dialyse away the ethanol and raise the pH to physiological pH, and you have your system that’s nice and stable.”
The other two lipid ingredients in that microfluidic whirlpool are phospholipids and cholesterol molecules, which can also be swapped out and tweaked to alter how LNPs behave and interact with the body. Thousands of different combinations have been engineered, tested and abandoned on the way to the LNPs in today’s vaccines. That said, as these LNPs come from a lineage originally optimised for expression in the liver, Cullis notes that there is plenty of scope to further improve their potency, stability and even cost. He points to the differing cold chain requirements of the Pfizer-BioNTech and Moderna vaccines to show that slight changes in formulation, or even just the time to do stability studies, can make profound differences. “If you look at the lipid constituents and the mRNA, they’re pretty much identical,” he notes. “And there’s no intrinsic reason why they can’t be more stable or more potent.” The CureVac candidate, for instance, which uses unmodified mRNA with similar LNPs, can be stored at 5°C.
Peer makes a similar point regarding manufacturing, noting that one of the systems he works with could create enough for the entire country of Israel in just 12 days. That’s still about 25 years for the world’s population, though – more systems are clearly needed than there were when mRNA drugs were a niche interest. Equally, he believes the more pressing bottlenecks in raw material supplies for synthesising lipids and generating RNAs are primarily a result of moving “from zero to 100 in a very short time”.
Open the door
Once they’re optimised for expression in a particular location, LNPs allow developers to simply swap out the genetic material they would like to deliver. That already makes them perfect for building vaccine platforms, as Moderna’s rapid responses to Covid-19 variants attest, but most LNPs are quickly removed from circulation and trapped in the liver, making it difficult to use them to address issues elsewhere in the body. Solving that might eventually mean sending the entire PEG clan to join their unnumbered cousins in the lipid family cemetery. In the same way they initially help LNPs sneak past the immune system, pegylated lipids inhibit their interactions with cells and can raise the risk of adverse reactions.
Still, PEG has a few more whirls through the bloodstream in it yet. In 2020, Peer’s lab made a breakthrough by using LNPs studded with targeting molecules to deliver mRNA encoding the CRISPR enzyme Cas9 to tumours in vivo, where, directed by guide RNAs also contained in the LNPs, it cut cancer cells’ DNA strands, effectively destroying them from the inside. It’s a similar ‘plug-and-play’ approach to the wider idea of LNP platforms, with a lipoprotein ‘universal adapter’ that binds on the lipid side to the LNP and on the protein side to specific regions of cells of the same isotype.
In mouse models, injected CRISPR-LNPs were selectively taken up by disseminated ovarian tumours, enabling up to ~80% gene editing in vivo, inhibiting tumour growth and increasing survival by 80%. Perhaps more impressively, intracerebral injection of cLNPs targeting the most aggressive types of brain cancer enabled up to ~70% gene editing in vivo, which caused tumour cell apoptosis, inhibited tumour growth by 50%, and improved survival by 30%.
Nonetheless, Cullis warns that more needs to be done for LNPs to effectively reach new locations in human bodies. The leaky vasculature of tumours means drug delivery systems diffuse into them more easily than other tissues, but sophisticated targeting systems count for nothing if the LNPs they decorate are quickly taken out of the bloodstream by the liver. “To get to other tissues, you have to have a very long circulation lifetime,” Cullis explains. GPS systems don’t compensate for a lack of fuel.
80%
Survival increase in mouse models with ovarian tumours treated with CRISPRLNP injections, which enabled up ~80% gene editing in vivo.
30%
Survival improvement due to intracerebral injection of cLNPs targeting the most aggressive brain cancers, which enabled ~70% gene editing in vivo.
Science Advances
“It’s all about timing in life. You need to be in the right location at the right time.”
Dan Peer
Though he’s wary of “simplistic thinking” about solving everything through targeting, Cullis believes the work that will open the rest of the body up to LNPs is already happening. “The door has been pried open a bit, we’ve got the crowbar in our hands,” he says. Neither he nor Langer imagined anything like what’s happened when they first tried putting drugs in nanocarriers, but, if anything, the next step sounds relatively simple for a technology that began by walking through walls. The work done by Peer’s lab to synchronise the expression of mRNA and guide RNA within cells in 2020 was a milestone for selective targeting in a year full of them. “It’s all about timing in life,” he observes wryly. “You need to be in the right location at the right time.” It might just take generations to get you there.